A long-running debate flared up again a few months ago on Twitter, when tech reviewer Ray Maker posted a comparison of three devices—from Stryd, Garmin, and RunScribe—that promise to measure how much “power” you’re generating during a run. “Anybody got any insights into why people think estimating mechanical power during running is useful?” asked Max Donelan, a neuromechanics professor at Simon Fraser University in Canada. “Unlike cycling, there is nearly no net external mechanical work.”
The answer, from someone at Stryd, one of the power meter makers, came swiftly and a bit peevishly: “Because it solves real problems.”
I’ve been following the rise of running power meters since 2014, when I first saw a Stryd prototype in action. It’s a neat idea, and one of the few genuinely new training aids in recent years. But I’ve had a really tough time getting my head around what running power actually means. Spurred by Donelan’s tweet, I’ve spent some time reading up on the concept and talking to people with widely diverging opinions on its practical usefulness. I don’t have an ultimate final answer on whether you should buy one, but I do have some thoughts on what a power meter is for runners, and what it’s not.
The Bike Analogy
To understand the potential appeal of running power meters, the best place to start is cycling. Bike-mounted power meters have been around since the 1980s. They measure the mechanical work you do in spinning the pedals by detecting the subtle bending of the cranks (or other parts of the bike, depending on the design of the meter you’re using). If you’re cycling at 250 watts, that means you’re doing 250 joules of work (about 0.06 calories) per second on the pedals, which primarily goes to overcoming air resistance (on flats and downhills) or gravity (on uphills).
Why is this useful to know? The golden promise made by power meters is that they give you a real-time measure of how hard your body is working. If you know that you’re capable of sustaining 250 watts for an hour, then you can use the power meter to keep your effort at that level even as your pace and heart rate rise and fall with hills and headwinds.
The implicit assumption here is that the watts you deliver to the pedals (the mechanical power) correspond to how hard your body is working overall and, more specifically, to the rate at which you’re burning food energy (the metabolic power). The metabolic power, after all, is what ultimately determines how quickly you’ll fatigue and whether you’ll exceed your lactate threshold or bonk. Normally, the only way to calculate metabolic power is to carefully measure the oxygen and carbon dioxide that you breathe in and out. That’s what the cumbersome mask and other equipment used during a VO2max test are for: to calculate metabolic power.
Humans are typically about 25 percent efficient at best, meaning that less than a quarter of our metabolic power gets converted into mechanical power, with the rest dissipated as heat. The precise relationship depends on exactly what your muscles are doing. Purely concentric contractions (a typical movement like lifting a weight or pressing down on a pedal) are the most efficient, at about 25 percent. Isometric contractions (where you exert force but your muscle doesn’t move, like pushing against a wall) consume metabolic power but don’t deliver any mechanical power. Eccentric contractions (braking motions like lowering a weight, where you’re pushing one way but moving in the opposite direction) actually have a negative efficiency of about -120 percent. There are also contributions from your tendons and other connective tissues, which can store energy like an elastic, then deliver mechanical power without consuming any metabolic power.
In other words, it’s complicated. If you’re cycling at 250 watts, it’s hard to know exactly how much metabolic power you’re consuming (or, to put it another way, how many calories you’re burning). It’s probably somewhere between 1,000 and 1,200 watts, but your pedal-mounted power meter can’t tell you the exact number. There’s a crucial saving grace for cycling power meters, though, which is that your efficiency stays roughly constant under all conditions, because pedaling a bike requires essentially the same motion on hills and flats, gravel and asphalt, in headwinds, and so on. Whatever your metabolic power is when the cycling meter says 250 watts, that relationship will hold true under all these conditions. The bottom line: In cycling, power is a good proxy for how much energy you’re burning.
The Trouble with Running
A power meter for running is attractive for exactly the same reasons as in cycling: as a real-time calorie estimator. So why has it taken so long for power to come to running?
Most of the discussions about running power focus on how much harder it is to measure compared to cycling. And this is true. In running, you have internal work (your arms and legs pumping back and forth) and external work (your center of mass bouncing up and down); you have positive work (pushing off with each stride) and negative work (braking as you land); and you have a significant contribution from elastic energy stored in your Achilles tendon and other tissues as they stretch upon landing and then snap back to help you push off. According to some (though not all) estimates, the energy stored and released in this way may contribute as much as half of the power required for each stride.
There’s no single device that can measure all these contributions. But the big technical advance of the past few years has been the arrival of wearable accelerometers and other sensors that can measure your stride and center-of-mass motion with sufficient detail to estimate a large fraction of this power. You can compare the readouts from these power meters to the gold-standard power measurements from laboratory-grade force-sensing treadmills that cost hundreds of thousands of dollars, and the agreement is pretty good. So, problem solved, right?
But the objection leveled by Donelan and other biomechanists isn’t really about measuring power; it’s about what power means. The underlying assumption is still that mechanical power during running is proportional to total energy consumption, as it is in cycling. But as obvious as that seems, it’s not at all clear that this is true. As one of the many papers on the topic argues, “measurements of whole body mechanical work cannot explain how the rates of metabolic energy consumption change with speed or animal size.” Or, as Donelan put it in a subsequent tweet: “The criticism of running mech[anical] power is not about how well current devices estimate it, but that even perfect measures are not closely related to effort in running.”
Stryd’s algorithm, as the company explained in a recent white paper, uses only the positive portion of mechanical power—how much you’ve generated to push off the ground. This seems reasonable on the surface, and the data (Figure 6 in the white paper) shows that this component has a nice tight correlation at various speeds with total energy consumption, which Stryd measures with VO2 equipment in a lab. In its dataset, Stryd’s power metric has a 96 percent correlation with total metabolic energy expenditure at different speeds—on a level treadmill.
Unlike in cycling, though, the relationship between mechanical power and total metabolic energy consumption doesn’t stay constant when conditions change. As you go up steeper and steeper hills, the contribution of elastic energy becomes increasingly negligible. At an incline of about 30 percent, you no longer have any bounce in your step. On downhills, your muscles do less positive work, and beyond about a 15 percent grade only do negative work, braking as you descend. Stryd’s conceptual shortcut, measuring only the positive mechanical power of your center of mass, can’t handle these changes and no longer has the same relationship with total energy consumption.
You might expect, then, that Stryd users would report crazy data whenever they venture into the hills. That doesn’t seem to be case—and the reason is simple. When I chatted with Stryd co-founders Kun Li and Wyatt Mohrman recently, they explained how their algorithm works. On level ground, as explained above, they estimate mechanical power from the motion of body’s center of mass, just like a force-sensing treadmill. But on hills, the relationship between mechanical power and energy consumption breaks down—so they reverse engineer it.
During the device’s development, they tested runners on inclined treadmills while wearing Stryd units, measured their oxygen and carbon dioxide consumption to calculate energy expenditure, and used that data to adjust their algorithm to give the “right” answers. So, on flat ground, they measured power (200 watts, say) and noted that this corresponded to a given rate of metabolic energy consumption (1,000 watts, say). On the inclined treadmill, they cranked up the speed and angle until the metabolic energy consumption was 1,000 watts, and then, for consistency, programmed the algorithm to call that power 200 watts.
From a scientific perspective, this means the number your running power meter spits out is essentially meaningless. Even on flat ground, “positive external mechanical power” is an indeterminate mishmash of contributions from muscles and springy tendons. It doesn’t accurately reflect the underlying processes that determine energy consumption. And on hills, you’re not looking at an actual power measurement at all—you’re looking at “the positive external mechanical power I would be generating on level ground if I burned energy at the same rate I’m burning it on this hill.” I don’t know the inner workings of the Garmin or RunScribe algorithms, but they face exactly the same issue: Either you’re measuring mechanical power or you’re estimating metabolic energy, but you can’t do both at once.
From a practical perspective, on the other hand, it’s totally fair to ask whether any of this really matters. Fundamentally, what Stryd and its competitors have built is a real-time metabolic energy consumption estimator—or, to be less fancy about it, a calorie counter optimized for running. It’s the equivalent of being able to carry around a full VO2 testing lab everywhere you run. Unlike heart rate, it responds instantly when you speed up or slow down. Unlike the pace on your GPS watch, it reflects the change in effort from going up and down hills. Unlike your internal sense of effort, it doesn’t (in theory) lie.
The Case Study
But does the disconnect between mechanical power and energy have any practical implications? Under certain circumstances, yes.
Consider, for example, some data collected by Hans van Dijk, a Dutch scientist who co-wrote The Secret of Running, on running with a power meter and optimizing running form. For cyclists, the power meter provides a useful tool for playing around with their riding position to look for efficiency improvements, so van Dijk and his colleagues tried a similar thing with running, asking a group of runners to increase or decrease their cadence by about ten steps per minute from their naturally chosen cadence. They measured efficiency in two different ways: once by measuring oxygen and carbon dioxide to calculate energy consumption, and once by measuring power using Stryd.
Here’s what that data looked like in trained runners:
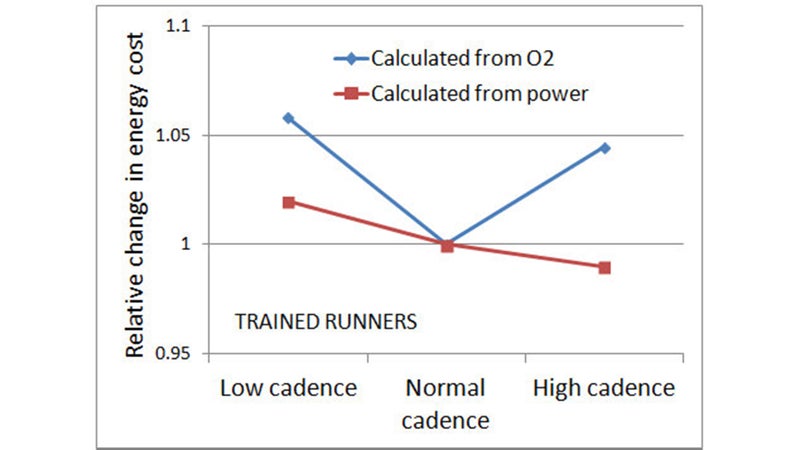
In this graph, a higher value means the runners had to burn more energy to run at a given pace. The blue curve, calculated from gold-standard oxygen and carbon dioxide measurements, shows the expected pattern. The runners were most efficient at their self-chosen cadence; increasing or decreasing cadence by about ten steps per minute forced them to consume more energy at the same pace—a bad thing.
But the red curve, calculated from Stryd’s power data, shows a different pattern. In this view, increasing cadence was a seemingly good thing, reducing power consumption and saving energy. When I asked Li and Mohrman about this data, they explained that it’s likely an artifact of the inability of the power meter to account for elastic energy stored and released by tendons. An ultrarapid cadence reduces up-and-down bounce in your stride, so the power meter thinks you’re getting more efficient. But it doesn’t realize that you’re losing out on some of the “free” energy return from your tendons.
The Bottom Line
At this point, you can probably see why I didn’t promise an “ultimate final answer” on the utility of power meters. Personally, I think tuning in to your internal effort sensor is both highly effective and really useful for racing to your limits. When I run, I don’t have any problems discerning whether I’m going up or down a hill. But I can also see the appeal of objective data that can help you pace yourself in uneven terrain and compare efforts on different courses where pace and heart rate aren’t reliable gauges.
For scientists, it will remain annoying that they’re calling this “power” at all, rather than something generic that doesn’t carry preexisting meaning (like, say, “NikeFuel”) and makes clear that it’s really intended as a real-time calorie tracker. But if, as the Stryd tweeter I mentioned at the top promised, the device ends up solving real problems for athletes, most people won’t lose much sleep about the nomenclature. Time will tell.
Discuss this post on Twitter or Facebook, sign up for the Sweat Science email newsletter, and check out my forthcoming book, Endure: Mind, Body, and the Curiously Elastic Limits of Human Performance.